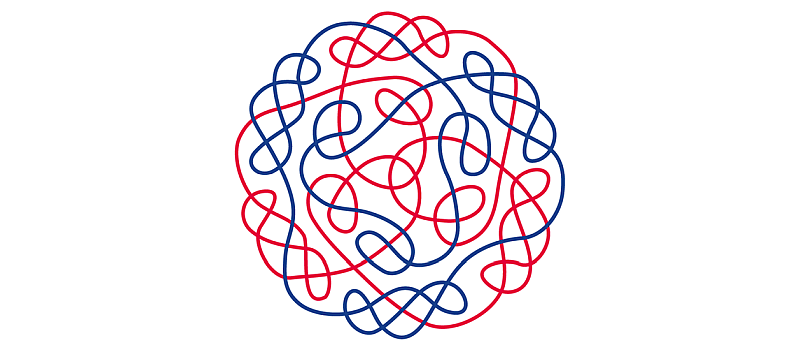
This blog was originally published on the Codasip blog. The more complex a processor core, the larger the area and power consumption. But increasing complexity is not a single dimension as processors can be more complex in different ways. In selecting a processor IP core, it is important to choose the right sort of complexity for your project. Some ways of thinking about complexity include:
- Word length
- Execution units
- Privilege/protection
- Virtual memory
- Security features